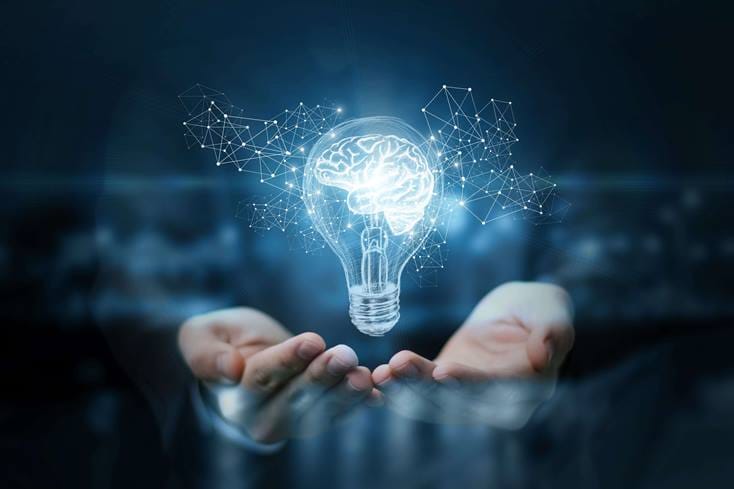
Technology is trending toward low-energy applications, including low-power networks, medical implants, wearables, robots, mobile devices, and more. Battery life is always a consideration in low-power devices, applications at a remote location, and medical implants. This article will examine smart energy management, including energy harvesting using sunlight, heat, and motion. Will it be possible to have a sustainable, renewable source that we can use in our daily lives and emergency situations?
The Problem of Energy Sourcing at the Edge
The growth of the internet of things (IoT) has been so robust that Cisco’s early prediction of 50 billion IoT devices by 2020 is looking increasingly feasible. However, the expansion of the IoT will cause logistics bottlenecks.
For example, there is a significant cost associated with the massive production of batteries. Also, the amount of raw lithium needed to produce enough batteries to power these IoT devices will far exceed annual global lithium production. In addition, the installation and replacement of these batteries for every IoT device will require tremendous, and possibly unscalable, costs, time, and labor. According to one calculation, in a world with 1 trillion battery-powered IoT devices and an average battery life of 10 years, there still will be more than 250 million IoT devices that need battery replacements every day. Moreover, the old, discarded lithium batteries present a significant burden to the environment.
That many of the devices will be installed in remote or hard-to-reach locations further adds to the challenge. Examples of remote locations include oil fields and wind turbines. Or take the case of a heart patient’s chest cavity, where doctors need to install and may later replace a pacemaker via surgery.
We can try to make batteries last longer by lowering the energy consumption of the devices. For example, the integrated circuits and sensors made with technologies such as Bluetooth Low Energy have ultra-low power consumption. Also, a “wake-up timer” that will trigger sensors to perform tasks within a short time frame only when needed can reduce power consumption by as many as 1,000×.
In addition, we can increase battery capacity. For example, working with other researchers, Nokia Labs has developed a new battery that promises to deliver 2.5× the longevity of today’s batteries.
Lastly, we can use inductive or wireless charging, transferring energy to the IoT device remotely. For example, the original implantable pacemaker was rechargeable. However, these rechargeable pacemakers required frequent replacement. Also, if the patient forgets to charge the pacemaker, the consequences could be life-threatening.
When all is said and done, improvements in energy efficiency or battery capacity do not address, but only delay, the inevitability of battery replacement.
In Search of Renewable Solutions
Therefore, battery-less IoT devices that can harvest energy from the surrounding environment are needed.
Solar Energy
Solar energy is an established technology, wherein solar radiation, or photovoltaic energy, is harvested and then converted into electric current. The main challenge for adapting solar energy for battery-less IoT will be miniaturization and flexibility.
Alta Devices had developed a solar-harvesting panel using a material called gallium arsenide. Its mini panels are said to be more efficient, thin, lightweight, and flexible than conventional panels. Alta Devices has applied its solar cells to power small, low-cost satellites containing electromagnetic, radiation, and inertial sensors for scientific analysis of the atmosphere. Other companies that have developed compact, low-power solar panels that can be installed on IoT devices include Voltaic Systems, ON Semiconductor, Ribes Tech, e-peas, EnOcean, and Everactive. Some of these companies also produce flexible panels that readily adapt to different IoT devices. Notably, Everactive can harvest solar energy even in dimly lit rooms.
Exeger, on the other hand, is taking another light-harvesting route. It uses a nano-material that, like chlorophyll in plants, converts photons into energy.
Thermal (Body) Heat
Today, more than 30% of the energy generated in the industry is lost through waste heat. Therefore, there is a significant amount of energy that can be harvested in industrial processes or cars.
There are several ways to harvest heat. Self-powered heating valves, enabled by a DC-to-DC converter and a Peltier element, need a temperature difference of only 2°C to deliver energy to electronic devices. Thermal energy can also be obtained through thermoelectric materials, such as crystals, which convert a constant temperature into electrical energy.
For example, The Face Companies’ Evercell thermal energy harvester leverages the temperature difference within a material to generate electricity, as long as the ambient temperature is above absolute zero. Other companies like e-peas, EnOcean, and Everactive have also developed a technology to harvest energy from temperature differences.
On the medical side, researchers are working on thermoelectric generators that can convert heat from the human body into a voltage to power medical sensors. Lastly, another lab is working on developing a pacemaker that is powered by parts of the human body that have a temperature difference of 5ºC.
Motion
The many ways to harvest energy from motion include mechanical energy, vibrational energy, and wave energy.
Mechanical energy is harvested through piezoelectric materials, which can generate electricity from pressure by accumulating an electric charge in response to mechanical stimulation. Dai Nippon Printing’s caster beacons are battery-less. The caster consists of an electromagnetic-induction–type rotary power generation unit and a beacon circuit. While the caster is rolling, it generates rotary energy to operate the beacon. Also, a group of researchers in China has developed a self-powered pacemaker that harvests energy from heartbeats. So far, the pacemaker has been tested on pigs only.
There is much vibration from the machinery in most industrial settings. Energy can be extracted from most vibrations and transformed into electricity by electrodynamic harvesters. For example, ReVibe Energy is developing vibration-powered sensors that have applications in aviation, industry, mining, and railway, where there are constant sources of vibration. Other companies like EnOcean, Everactive, and e-peas have also developed products to harvest vibrational energy.
A piezoelectric approach can also enable energy extraction from the ocean’s tides for conversion into electricity.
Building a piezoelectric device into an underwater pump of an offshore oil rig makes it possible to harvest energy from waves. The harvested energy can be used to power IoT sensors. This way, an IoT sensor that monitors the pump status can be powered to collect and transmit data. A research lab at MIT also used the piezoelectric approach to develop a battery-free sensor for underwater exploration.
Radio Waves and TV Signals
Radio waves are a type of electromagnetic radiation. Electromagnetic energy is abundant in space, coming from various sources such as satellite stations, wireless internet, radio stations, and digital multimedia broadcasting.
A power system that harvests radio-frequency energy consists of an antenna and a rectifier circuit. The harvesting system can capture and convert electromagnetic energy into alternating electric current or direct current.
Wiliot’s battery-free Bluetooth tags can also harvest energy from ambient radio-frequency energy in the surrounding environment. Everactive has also developed similar products. Lastly, e-peas has developed technologies to scavenge energy from various radio-frequency sources.
Wireless Charging
If energy harvesting from the surrounding environment is not feasible, the transmission of energy from an external source to the IoT device may be an alternative. For example, Wi-Charge’s compact, long-range wireless charger can be plugged into standard electric sockets and then used to transmit energy to IoT devices that are as far away as 30 feet. These remote wireless chargers will eliminate the need for power cords as well as battery replacement.
The effort to develop pacemakers that can be wirelessly charged, noted earlier, recently got a significant reboot. A collaborative team from Rice University and the Texas Heart Institute has developed a battery-less pacemaker that harvests radio-frequency energy that is being transmitted by an external battery pack. This strategy can sidestep the previous need to replace an aging battery via surgery. Also, this method overcomes the problem of the patient forgetting to charge the pacemaker, because an external mechanism can now control transmission of radio-frequency energy to the pacemaker.
Future Trends and Challenges Ahead
Batteries have been around for many years. Going battery-free has long-term benefits and sustainability, but it would require upfront investment in implementing energy-harvesting technologies. Additionally, there are potential issues that need to be addressed.
While we can try to select the most consistent source of energy for harvesting in the battery-less system, we still need to prepare for potential energy outages. Therefore, the battery-less IoT system needs to be as energy-efficient as possible. For example, a wireless communication platform from Jeeva Wireless has reduced power by 10,000× compared to conventional radios. Also, we need smart sensors to analyze the energy necessary to complete each sense-transmit cycle and the repeat interval required; this way, we can ensure that enough power is harvested to perform the cycle.
Another challenge will be to improve the longevity and performance of the IoT devices’ non-battery components. Efforts to increase battery longevity, particularly for pacemakers, did not gain traction because there was no point in developing a battery that outlasted the other components; a surgery would be needed to replace the entire system as soon as one component became defunct. Therefore, it is critical to make sure the progress made in the power supply system closely matches that made in the rest of the IoT device.